Innovative Breakthroughs: How Universities Drive Invention
Written on
Chapter 1: The Crucial Role of Universities in Innovation
In the realm of innovation, the journey to success often spans decades, with universities serving as the foundational incubators for many groundbreaking technologies.
This passage highlights how significant breakthroughs are often the result of years of research and development within academic institutions.
Section 1.1: Historical Foundations of Technological Success
Almost every major technology that eventually transforms industries and society originates from universities, typically requiring extensive time to mature. The Internet, for instance, was established among universities in the late 1960s but only gained widespread adoption in the 1990s. Similarly, artificial intelligence, which has become an integral part of modern life, traces its roots back to university research in the 1950s.
Tony Peacock, a former CEO of Australia’s Cooperative Research Centres Association, noted, “Many pivotal Australian scientific advancements, such as the HPV vaccine and the Cochlear implant, are deeply embedded in university research.” These innovations, which enhance hearing and combat cervical cancer, exemplify the vital contributions of academic research.
Additionally, success stories from the Commonwealth Scientific and Industrial Research Organisation (CSIRO) — including Wi-Fi and polymer contact lenses — underscore the importance of collaboration between universities and research institutions like Macquarie University and the University of New South Wales.
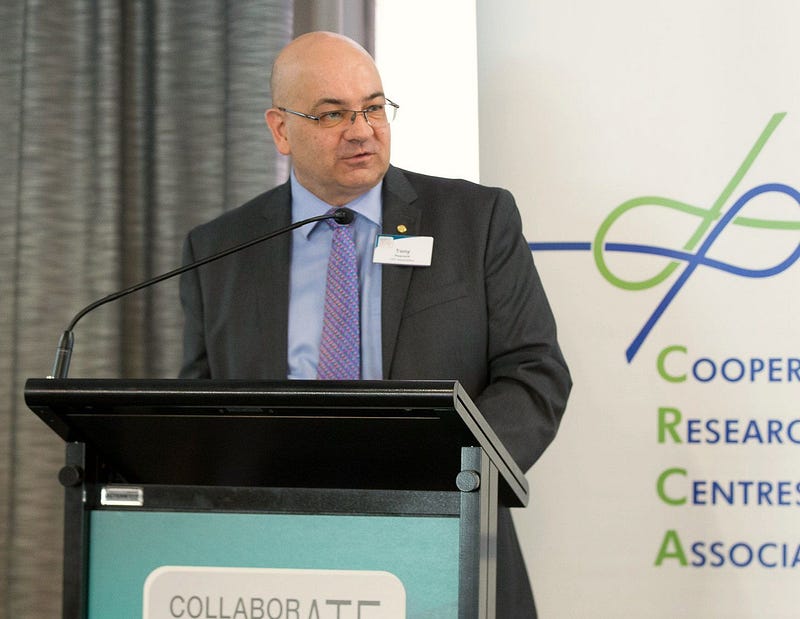
Section 1.2: A Global Perspective on University Research
The influence of universities on innovation extends beyond Australia. “History is filled with instances where fundamental research conducted at universities, driven by researchers’ curiosity, led to significant applications,” remarked Kate Smith-Miles, a Professor of Mathematics and Statistics at the University of Melbourne. She emphasized the mathematical breakthroughs that enabled the development of the Internet and MRI technology, as well as the foundational understanding of DNA that made modern sequencing and genetic engineering possible.
Prof Caroline McMillen, Chief Scientist for South Australia, highlighted the necessity of collaboration among various stakeholders to create a robust value chain for innovation. She referenced examples from cities like Albany, New York, and Eindhoven in the Netherlands, which transformed old industries into modern, tech-driven enterprises through partnerships involving local universities, government initiatives, and startups.
“Communities experienced remarkable economic and social shifts as traditional manufacturing declined,” McMillen explained. “This created a pressing demand for new ideas, and they turned to universities for solutions.”
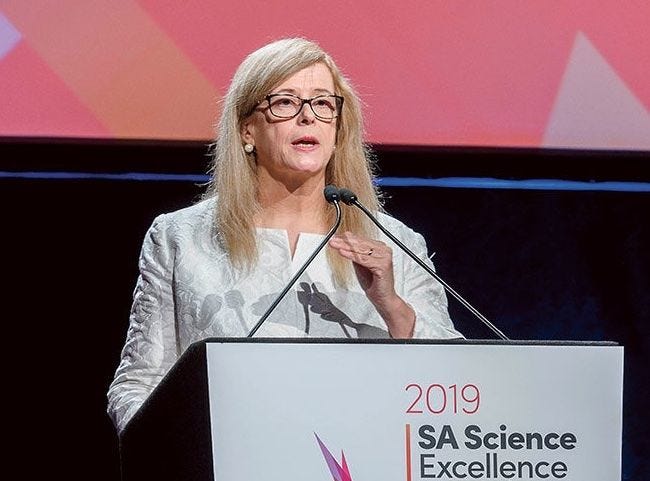
Section 1.3: Transforming Industries Through Collaboration
Take Akron, Ohio, once the heart of the tire manufacturing industry with companies like Goodyear and Firestone. McMillen noted that the region’s expertise in polymers prompted a shift to explore applications beyond traditional uses of plastics and rubber. From the economic downturns of the 1990s, Akron has now emerged as a leading center for polymer innovation, with over 400 companies engaged in producing polymer-based materials for various industries, including cosmetics and medical devices.
In Australia, progress in this area has been more gradual, partly due to businesses being cautious and less familiar with university partnerships. However, this dynamic is evolving. “University practices around intellectual property management and external collaborations are still catching up to international standards,” Peacock observed. “Yet, researchers are increasingly adept at engaging with businesses, moving beyond viewing companies merely as funding sources.”
Despite universities being essential links in the innovation value chain, many Australian companies have yet to recognize their potential. “However, awareness is growing regarding the advantages universities can offer,” Peacock added. “Often, initial interactions stem from a simple task that requires assistance, which can lead to more meaningful collaborations.”
“There’s no singular approach to collaboration,” McMillen pointed out. “It all commences with a dialogue.”
Manufacturing the Future
Universities function as the epicenters of innovation, where numerous novel ideas emerge each year. Some examples include...
The last five decades have witnessed a remarkable surge in technologies emerging from basic research, significantly impacting the world. From the advent of artificial intelligence in 1956 to the development of the World Wide Web in 1989, and the introduction of CRISPR-Cas9 gene editing technology in 2012, these innovations have transformed industries and society alike. What groundbreaking technologies might lie ahead?
One promising avenue is messenger RNA (mRNA) technology, initially developed in the 1990s. This technology played a vital role during the COVID-19 pandemic by enabling the rapid production of tailored proteins, revolutionizing drug development processes and paving the way for new treatment possibilities.
Another exciting field is quantum sensing, which leverages the principles of quantum mechanics to achieve unparalleled precision in measuring physical phenomena. Experts predict that this technology will lead to significant advancements in imaging, navigation, and our understanding of biological systems.
Collaborative projects, such as the Square Kilometre Array in radio astronomy, spanning Australia and South Africa, are generating vast datasets that will necessitate innovative technologies for processing and analysis, ultimately benefiting society at large. Real-time data processing systems are already being employed commercially, aiding in rapid stock market analysis and enhancing medical diagnostics.
However, two pivotal technologies poised to reshape the future are synthetic biology and quantum computing.
Chapter 2: The Evolution of Synthetic Biology
Synthetic biology merges biology, engineering, and computer science to design and construct artificial biological systems or re-engineer existing ones. This field is accelerating advancements in biotechnology across industrial and medical sectors, fostering sustainable manufacturing and energy production methods. Potential applications include biofuels, innovative vaccines and therapies, and improved agricultural resilience against pests and climate change.
The global synthetic biology market was valued at approximately US$14.15 billion in 2021 and is projected to reach US$45.7 billion by 2026. Australia’s Synthetic Biology Roadmap anticipates that synthetic biology could generate A$27 billion annually for the country and create 44,000 new jobs by 2040.
Prominent Australian companies in this space include Samsara Eco, which employs enzyme-based technology to decompose plastic, and Starpharma, which utilizes nanoscale polymers for targeted drug delivery. Leading academic institutions in Australia include the University of Melbourne, Monash University, the University of Sydney, Macquarie University, the University of Queensland, and UNSW.
Timeline of Synthetic Biology Developments:
- 1953: Discovery of the double helix structure of DNA at the University of Cambridge.
- 1972: Development of recombinant DNA technology at Stanford University and UCSF.
- 1977: Sequencing of the first complete genome at the University of Cambridge.
- 2010: Creation of the first synthetic cell at the J. Craig Venter Institute and MIT.
- 2022: Development of the first fully synthetic yeast genome by Macquarie University and collaborators.
Chapter 3: The Future of Quantum Computing
Quantum computing is an emerging field that harnesses the principles of quantum mechanics to create powerful computational systems. Unlike classical computers, which operate using binary bits (0s and 1s), quantum computers utilize quantum bits (qubits) that can exist in multiple states simultaneously. This phenomenon, known as superposition, enables unparalleled computational capabilities.
Another key aspect is entanglement, which allows quantum computers to process data concurrently. Although still in its infancy, quantum computing is already being used to simulate chemical and molecular interactions, aiding in the discovery of new drugs and materials.
As quantum technology matures over the next decade, it is anticipated to revolutionize fields such as cryptography, financial modeling, and chemical engineering, addressing complex optimization challenges like scheduling and routing, and accelerating machine learning algorithms.
The global quantum computing market is projected to reach US$186 billion by 2030, with an estimated A$2.2 billion in Australian revenue and the creation of nearly 19,400 new jobs by 2045.
Prominent Australian enterprises include PsiQuantum, focused on developing silicon photonics-based quantum computers, and Silicon Quantum Computing, which works on single-atom qubits. Leading universities in this field encompass UNSW, the University of Sydney, the University of Melbourne, Monash University, and the University of Queensland.
Timeline of Quantum Computing Developments:
- 1980: Proposal for using superposition and entanglement in computation at Moscow State University.
- 1994: Peter Shor devises a quantum algorithm that can factor large numbers rapidly, threatening current cryptographic systems.
- 2001: The first implementation of Shor’s algorithm occurs at Stanford University.
This article first appeared in Australian University Science, March 2023.
Like the story? Please hit the ? button below, or ‘follow’ at the top.
? Support my writing by joining Medium ?